Multi-Scale Modeling of Battery Physics
NREL's physics-based electrochemical models accelerate the development of new and improved battery materials, designs, and algorithms for various grid and transportation applications.
In coordination with digital twin models for system lifetime and control, the NREL's multi-scale model portfolio greatly reduces the time and cost of battery research and development cycles. These models guide the optimization of energy- and power-dense batteries for applications ranging from electric vehicles to electric aircraft.
Capabilities
NREL uses coupled electro-chemo-thermo-mechanical models to understand the performance and degradation pathways of batteries under various operating conditions. Models optimize many aspects of battery design, including active material and electrode architecture, 3D cell and cooling system design, and mechanical constraints and packaging.
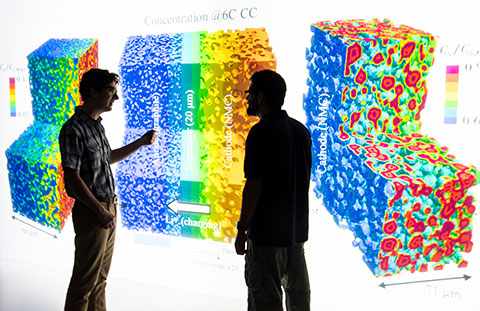
Researchers discuss simulation results from NREL's Microstructure Analysis Toolbox.
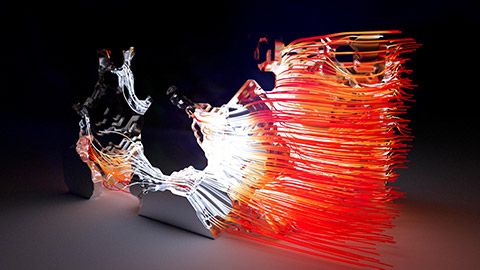
This model shows flux of Li-ions moving through a battery from the electrolyte into the negative electrode. Bright parts depict high flux as the ions must converge and travel faster through constricting pores. Visualization by NREL
NREL's multiscale computer-aided engineering tools help battery designers create the advanced battery technologies needed to boost battery performance. By coupling physics across different length scales, these models can predict nonuniform reactions, temperature, and stress hotspots that lead to early failure.
Cell- to pack-level models pair reduced-order electrochemical submodels with high-fidelity 3D thermal and mechanical models to quantify heat flow, electron flow, and stress characteristics. These models aid in pack optimization and include auxiliary systems such as cooling fluids/channels, stack loads, and mechanical constraints.
High-fidelity electrode- to cell-level models unravel coupled thermodynamic, kinetic, and transport phenomena occurring inside porous electrodes. They provide a detailed picture of species, charge, and heat motion through the electrode and electrolyte phases and are well suited for beginning-of-life battery design, including optimization of energy, power density, and fast charge.
Microstructure models, such as the Microstructure Analysis Toolbox, are based on 3D geometry of active material particles packed into the composite electrode. These models quantify the tortuosity, or distance that ions must travel, as well as the constrictions they face as the ions move across the electrode thickness. Such fundamental insights are useful in optimizing particle and pore architecture. These models link manufacturing processes with desirable structures and how those structures impact performance.
Data-driven reduced-order models incorporate advanced statistics and machine learning to diagnose and predict battery cycle and calendar aging (respectively, energy and time throughput). Trained with accelerated-aging test data, NREL's lifetime models predict battery life and how it varies under conditions such as charge/discharge rate, ambient temperature, and state-of-charge history. These models map data and usage conditions to lifetime outcomes, which can approach millions of miles and decades of battery lifetime.
NREL researchers are developing advanced chemistry models for next-generation materials such as silicon, lithium metal, solid electrolytes, and sulfur. These models include complex reaction pathways, evolving interfaces, and large deformations to capture the strong coupling between reaction kinetics, transport phenomena, and stress mechanics. These models enhance our fundamental scientific understanding of next-generation, novel-chemistry systems.
Applications
Model-guided optimization accelerates the development of next-generation battery designs to meet fast-charge, high-energy, low-cost, and long-lifetime performance goals.
To compete with refueling times of internal combustion engine vehicles, 10- to 15-minute fast charging for electric vehicles is needed. NREL's suite of micro-to-macro models aids in rectifying inherent reaction and transport bottlenecks in lithium-ion cells. Models optimize costs and benefits of disparate fast-charge technologies ranging from polycrystalline active-material architecture, electrode structures, advanced electrolytes, thermal strategies, and charge protocols to guide 3D system design and manufacturing requirements.
Degradation models measure the effect of key degradation mechanisms, such as lithium plating or cathode cracking, on the battery's overall performance. Researchers use degradation models to compare reactions within the battery to evaluate new battery materials for improved performance, lifespan, and safety. Models coupled with artificial intelligence diagnose degradation mechanisms from electrochemical and microscopy experimental data.
Solid-state batteries combine high-capacity anodes, such as lithium and silicon with solid-state electrolytes, to significantly increase battery energy density. Solid-state batteries also enhance safety by eliminating flammable liquid electrolytes. NREL's solid-state models provide a map of mechanical phenomena (stress concentrations, plastic flow, delamination) coupled to electrochemistry. These models help develop strategies to reduce the need for external stack pressure and enhance performance and lifetime.
NREL's Lithium-ion Battery Resources Assessment (LIBRA) model is used to analyze future scenarios governing the buildout of the global supply chain for critical lithium-ion battery materials. LIBRA tracks the flow of these materials while using techno-economic structures to determine the impactful factors that vary investment in manufacturing and recycling facilities, given variations in model inputs such as battery demand and the resulting recycling feedstock composition.
Publications
Electrode Scale and Electrolyte Transport Effects on Extreme Fast Charging of Lithium-Ion Cells, Electrochimica Acta (2020)
Laser Ablation for Structuring Li-Ion Electrodes for Fast Charging and Its Impact on Material Properties, Rate Capability, Li Plating, and Wetting, Journal of Power Sources (2022)
Resolving the Discrepancy in Tortuosity Factor Estimation for Li-Ion Battery Electrodes Through Micro-Macro Modeling and Experiment, Journal of The Electrochemical Society (2018)
MATBOX: An Open-Source Microstructure Analysis Toolbox for Microstructure Generation, Segmentation, Characterization, Visualization, Correlation, and Meshing, SoftwareX (2022)
Quantifying the Influence of Charge Rate and Cathode-Particle Architectures on Degradation of Li-Ion Cells Through 3D Continuum-Level Damage Models, Journal of Power Sources (2021)
Lithium-Ion Battery Life Model With Electrode Cracking and Early-Life Break-In Processes, Journal of The Electrochemical Society (2021)
Machine-Learning Assisted Identification of Accurate Battery Lifetime Models With Uncertainty, Journal of The Electrochemical Society (2022)
A Reformulation of the Pseudo2D Battery Model Coupling Large Electrochemical-Mechanical Deformations at Particle and Electrode Levels, Journal of The Electrochemical Society (2019)
Enabling Extreme Fast-Charging: Challenges at the Cathode and Mitigation Strategies, Advanced Energy Materials (2022)
Influence of Metallic Contaminants on the Electrochemical and Thermal Behavior of Li-Ion Electrodes, Journal of Power Sources (2022)
Contacts
Share
Last Updated March 3, 2025