Engine Combustion Research
NREL's engine combustion research focuses on fuel efficiency, engine performance, and net-zero emissions. It spans from reciprocating engine combustion to combustion mechanism and kinetics.
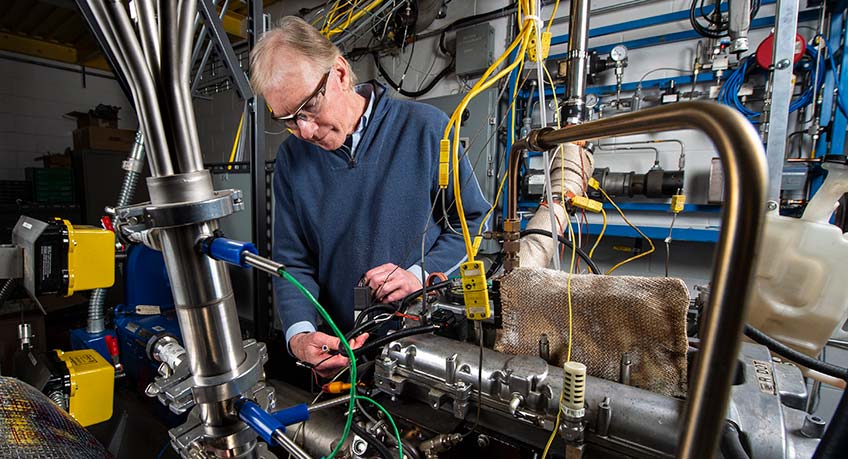
Reciprocating Engine Combustion
Our research uses actual reciprocating engines, which allows us to quantify the effects of fuel properties and fuel chemistry on engine performance and exhaust emissions. We then use the results to validate predictive models or gather insights leading to improved models.
Machine Learning
NREL is a world leader in using machine-learning techniques to augment combustion models and develop combustion control strategies. These strategies can effectively be used to develop design tools and guidance that enable the aviation industry to craft next-generation turbine and engine component designs that can operate on 100% sustainable aviation fuels.
Single-Cylinder Engines
We operate single-cylinder engines for both spark-ignition and compression-ignition (diesel) combustion. A single-cylinder engine allows researchers complete control over all engine combustion parameters, such as injection timing, intake pressure, and exhaust gas recirculation. Fuel impacts—and the interaction of fuels with engine combustion parameters—can be assessed.
Heavy-Duty Multicylinder Engine Dynamometer
We conduct experiments using a heavy-duty multicylinder engine dynamometer to evaluate fuel effects on original equipment manufacturer production engines over transient cycles, including assessment of impacts on emission control catalysts and filters.
Publications
Read about related studies and browse all NREL publications on engine combustion R&D.
Effects of Heat of Vaporization and Octane Sensitivity on Knock-Limited Spark Ignition Engine Performance, SAE International (2018)
Impact of Ethanol Blending into Gasoline on Aromatic Compound Evaporation and Particle Emissions From a Gasoline Direct Injection Engine, Applied Energy (2019)
Contact
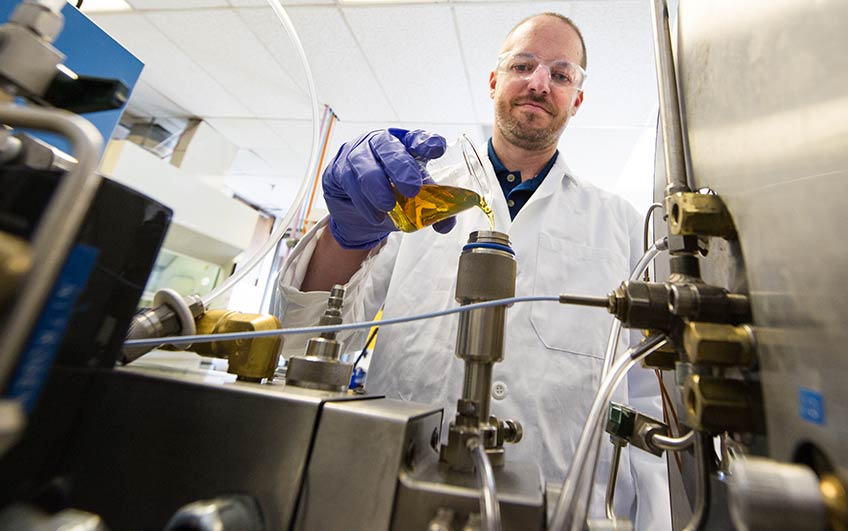
Engine Combustion Mechanism and Kinetics
Through fundamental kinetics and high-fidelity combustion simulations, we work to form a molecular-level understanding of fuel property impacts on engine efficiency and emissions. We leverage high-performance computing resources to allow for the rapid development and market entry of net-zero-carbon on- and off-road transportation fuels that are compatible with existing engines, enabling the reimagination of future efficient engine design.
Laminar Flow Reactor for Kinetic Simulations
For development of accurate kinetic simulations, NREL uses a laminar flow reactor to study mechanisms of fuel autoignition and soot precursor formation by quantification of stable intermediates. The reactor consists of a:
- System for metering gases and fuels
- Stainless steel with inert coating
- Gas sampling and analysis system.
Advanced Fuel Ignition Delay Analyzer
NREL is using the Advanced Fuel Ignition Delay Analyzer to improve the homogeneity of the fuel-air mixture and quantify the degree of mixing with computational fluid dynamics simulations for a fuel chemical kinetic mechanism experiment.
For shorter ignition delay times, the developed computational fluid dynamics model has been shown to accurately separate the chemical and physical effects. The instrument can provide high-quality ignition delay measurements, especially for the critical low-temperature and negative temperature coefficient ignition regions.
NREL is determining how this experiment can be used to describe the combustion quality of jet fuels more thoroughly compared to a traditional single cetane number measurement, which may help speed up the introduction of sustainable aviation fuel into the market.
Publications
Read about related studies and browse all NREL publications on combustion mechanism and kinetics R&D.
Chemical Kinetic Basis of Synergistic Blending for Research Octane Number, Fuel (2022)
Elucidating the Chemical Pathways Responsible for the Sooting Tendency of 1 and 2-Phenylethanol, Proceedings of the Combustion Institute (2021)
Contact
Share
Last Updated March 4, 2025