Opportunity for Chemicals and Fuels From Carbon Dioxide: Researchers Assess Roadblocks for Industrial Deployment of CO2 Electrolysis
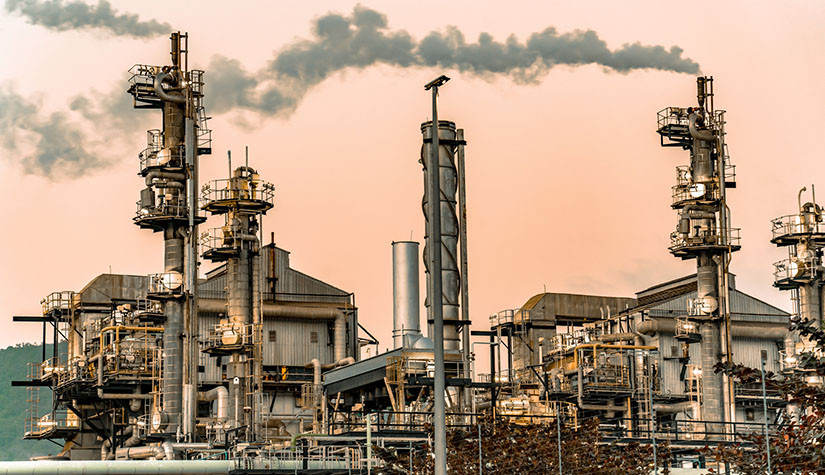
Zero-emission energy systems have a gigaton-sized challenge: Waste carbon dioxide (CO2) will continue to flow into the atmosphere as long as steel, cement, and chemical plants exist. These and related industries are hard to decarbonize, but with upcoming technologies, waste CO2 could potentially be captured and converted into petrochemical products and fuels. In a perspective published in Joule titled Barriers and opportunities for the deployment of CO2 electrolysis in net-zero emissions energy systems, the U.S. Department of Energy's National Renewable Energy Laboratory (NREL) and collaborating authors holistically review the status of CO2 electrolysis technology and its potential to reduce carbon emissions in future energy systems.
Electrolysis uses electricity—in this case from renewable and low-carbon energy—to reduce CO2 into chemical commodities, notably carbon monoxide, ethanol, formic acid, and ethylene. Although CO2 electrolysis is scarcely in use today, its technical maturity and economic appeal are improving, prompting the authors to scrutinize the factors surrounding its deployment and integration with clean-energy and carbon-capture technologies.
"Despite recent progress on lab-scale technology performance, roadblocks for the industrial deployment of CO2 electrolysis remain," said Omar Guerra Fernández, lead author and NREL grid researcher. "We set out to determine how electrolysis could contribute to a net-zero-emission energy system and what is needed to make it happen."
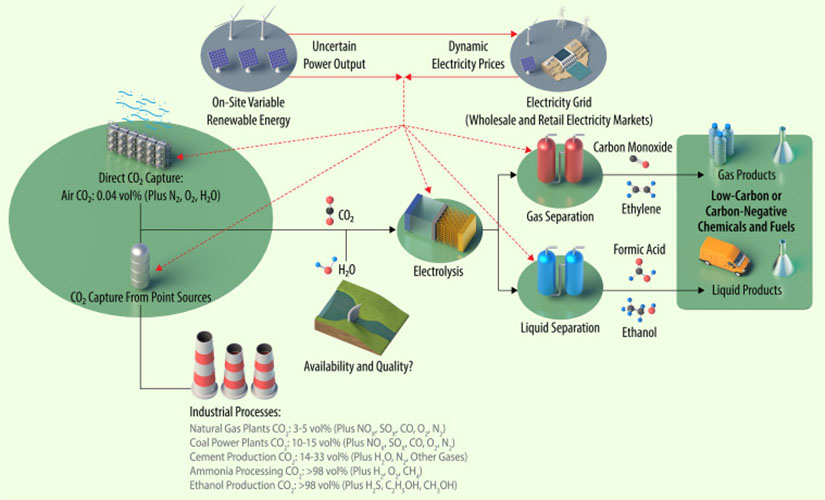
High-Level Findings From the Study
Despite recent progress in component design and mechanistic understanding, CO2 electrolysis remains a fringe technology. Its relatively high capital and operating costs are not yet competitive with conventional production processes. However, this is contingent on electricity pricing and markets, policy, and performance of electrolyzer components. The study thoroughly analyzes how each component—catalysts, membranes, and reactors—and each integration pathway affects the economic feasibility of CO2 electrolysis.
The authors find that the source of waste CO2 is highly relevant to whether electrolysis could be cost competitive. For example, ammonia plants provide consistent, high-proportion CO2 waste, but not at a substantial volume compared to cement plants, which the authors identify as a possible steady and reliable source of CO2 for electrolysis. In addition, impurities from CO2 waste streams (e.g., nitrogen oxides) require further separation steps that could increase the total process integration cost.
Another major finding is that renewable electricity could take CO2 electrolysis a long way toward cost competitiveness. By selectively taking advantage of variable electricity from wind and solar resources, CO2 electrolysis could benefit from low-cost electricity while also providing a flexible load. Potentially the most viable pathway combines on-site renewable energy with grid electricity and flexible electrolyzers; however, further analysis will need to determine the full economics of cycling electrolyzers with variable renewable power generation and dynamic electricity prices.
Where Work Is Needed for CO2 Electrolysis
Undoubtedly, the most effective way to advance CO2 electrolysis is for early adopters to deploy, share lessons, and iterate. With real examples underway, industry can get crucial data about component durability and pathway efficiencies. Even run time in a laboratory setting can help elucidate the potential value of CO2 electrolysis, as thousands of hours of operation are needed to accurately understand how electrolyzers degrade.
Among the electrolyzer components, tolerance to impurities is an important line of research. Nitrogen oxides and sulfur oxides are regular constituents of waste streams that reduce electrolysis efficiency. While direct air capture allows purer CO2 intake, the authors find that costs are not currently justified.
Similarly, trade-offs exist in efficiency depending on the chemical that is being manufactured. For example, formic acid has a relatively high market price and CO2-to-product conversion rate but has lower market demand. Trade-offs also exist around which catalysts are best matched for specific products. More use data can resolve those tradeoffs in greater detail.
As for integration with renewable energy, use data will reveal whether electrolyzers can tolerate the frequent cycling associated with variable power and how the economics appear under various integration pathways.
The Takeaway
It is estimated that in 2050, 7.6 gigatons of CO2 will need to be captured to achieve net-zero energy globally. The hardest sectors to decarbonize will likely rely on some form of carbon capture that can be integrated with CO2 electrolysis to produce carbon-neutral or carbon-negative commodities using clean power.
Industry adoption could quickly bring CO2 electrolysis to maturity by helping operators understand the economics and technical impacts of electrolyzer deployment. Of particular interest, integration with renewable energy and CO2 capture could provide cost benefits that are largely unexplored.
Learn more about NREL's energy analysis research.