NREL Authors Publish Quantum Computing First in Nature Communications
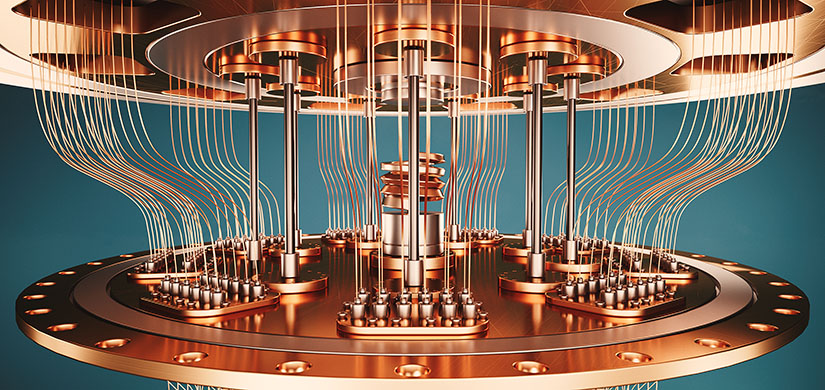
In years ahead, quantum computers are anticipated to explore beyond what is possible with classical computers. This could hold true for studying complex systems, which manifest in everything from our brains to the power grid, and often max-out the abilities of supercomputers when being simulated.
The first complexity analysis with a quantum computer has now been achieved by a team of scientists, including two from the National Renewable Energy Laboratory (NREL), who have implemented quantum cellular automata on a 23-qubit quantum computer. Their paper, “Small-world complex network generation on a digital quantum processor,” was published in the journal Nature Communications.
Physical complexity appears throughout nature and is indicated by qualities like spontaneous pattern formation and self-organization. For example, researchers at NREL encounter complexity in areas like nanoscale materials and urban infrastructure. But, true to the name, complexity can be tough to study. Scientists expect that quantum computers—which use a fundamentally different mode of calculation than classical computers—might also represent complexity in a fundamentally different, more useful way. This publication showed that complexity can in fact be simulated on a quantum computer—a first for the field and an important step toward future complexity analysis.
Although simulating a brain is certainly not an option in these early days of quantum computing, complexity can still emerge from relatively simple algorithms. For this study, the authors turned to cellular automata, a class of elementary rules that famously generate lifelike systems. The question for the research team was, could a quantum version of cellular automata display complexity on a quantum computer?
Structure From Simplicity
Cellular automata are a heady toy for computer scientists. Automata are modeled on a lattice, where each square is either black or white (1 or 0, or up and down, if you prefer). The squares update their state according to the state of neighboring squares. Simple as it sounds, there are many different rules that lead to unique and astonishing structures—some that evolve indefinitely, others that are genuine computers. From this vast field, the authors identified “Goldilocks rules,” cellular automata that are not too active, not too passive, just right to reproduce common features of complexity. Too active would lapse into chaos, while too passive would fizzle into triviality.
The blue diamond structure shows persisting coherence among the qubits as the quantum cellular automata (QCA) algorithm runs. The emulated data are from a classical computer emulation of the experiment, the raw data are unfiltered results from the experiment, and the post-selected data show experimental results following post-selection treatment.
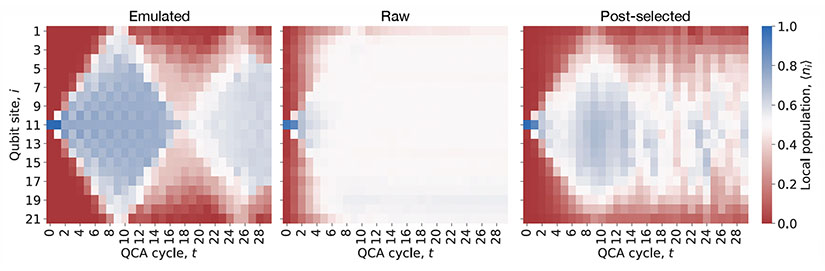
Starting with one qubit “up” and the rest “down,” the scientists let their circuit evolve and periodically measured the qubits. The scientists then artfully filtered the data to reveal an underlying structure that persisted throughout the program runtime—a diamond pattern of qubit correlations that resisted the trend toward randomness. This was their stamp of quantum complexity.
To measure the degree of complexity, the scientists borrowed a metric used in neuroscience named mutual information, which quantifies the amount of correlation among qubits. From their analysis, the scientists found that the qubits evolved in a self-organized pattern that retained order for a significant number of time cycles. Like brains or friend groups, the correlations between qubits indicated the formation of “small-world networks,” which are networks that exhibit high connectivity and short path length between nodes. Basically, one qubit’s evolution is tightly connected to the evolution of others.
Applications and the Origins of Complexity
The team’s results are an experimental landmark for quantum computing. The research provides a template for how to investigate quantum cellular automata—an expansive field for future study—and a starting point to investigate complexity with quantum computers. Future applications might use analyses with 20,000 qubits, rather than 20, to simulate highly correlated complex systems. The hope is that qubits might replicate some of the deep entanglement seen on complex networks in a truer way than computers currently can.
Potentially even more profound, the researchers are striking at the origins of complexity itself. Complexity emerges ubiquitously in nature, and the quantum scale is no exception. This work provides an experimental validation of quantum complexity and can begin to ask how dynamics at that scale relate to the complexity we see every day.