NREL Chemist Wins Award for Electron Bifurcation Research Article
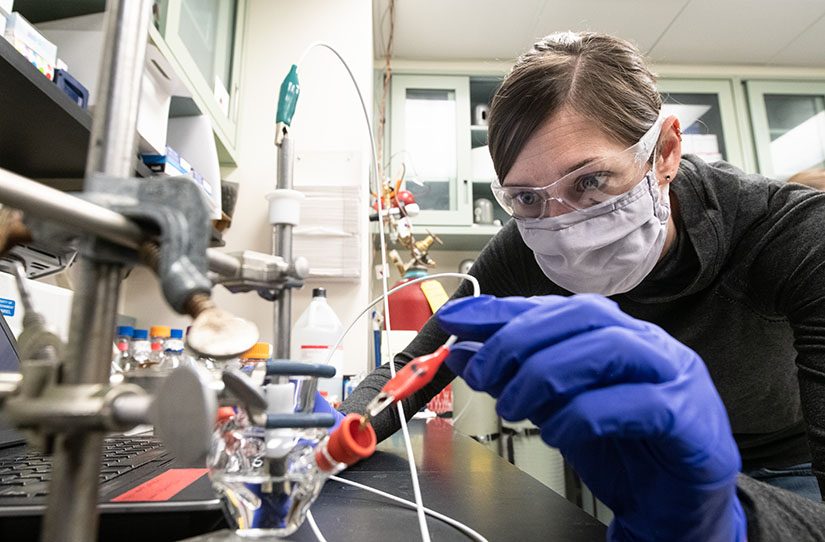
Cara Lubner, a chemist at the National Renewable Energy Laboratory (NREL), coauthored a research article on electron bifurcation that was awarded the Class I: Physical and Mathematical Sciences 2020 Cozzarelli Prize on April 25.
Of the 4,000 research articles published in the Proceedings of the National Academy of Sciences of the United States of America (PNAS) journal last year, “Universal free-energy landscape produces efficient and reversible electron bifurcation” was one of only six articles to receive this award for “exceptional scientific achievement.” This work was part of Lubner’s recent Office of Science Early Career Research Program award.
The purpose of the research was to understand how certain organisms use electron bifurcation to control energy in an efficient and specific manner. By combining experimentation and theory, and building upon prior scientific findings, throughout the research process, her team generated fundamental knowledge about this mechanism that will inform future work.
“The article by Cara and her collaborators reveals how biology has many surprises yet to be discovered, and the inspiring research being conducted by her team is likely to foster the design of new synthetic concepts for directing electron flow to achieve highly efficient chemical reactions,” said NREL Principal Scientist Paul King.
Lubner hopes that as more people learn about this work they will appreciate how “amazing” the organisms that conduct electron bifurcation truly are.
“This mechanism is used throughout most domains of life, and it’s the epitome of what we’re trying to do,” Lubner said. “It can perform chemistry really well, it’s super selective and efficient, and I see it as such an important target to better understand so that we can take those principles and apply them to other aspects of science that don’t have to do with biology.”
Electron Bifurcation: It Boils Down to Vegetables and Dessert
Energy conservation is an essential feature of life that allows organisms to exploit their surroundings and grow more efficiently and productively than other organisms. Electron bifurcation—a mechanism where an enzyme uses a pair of electrons in a highly controlled manner—is a method many organisms use to conserve energy.
When an enzyme utilizes electron bifurcation, it creates a product that has more energy than the material it originated from.
For years, scientists have studied this phenomenon—Lubner’s team built on research dating back to the 1970s. She said one of the reasons this method is intriguing is because, on its surface, it appears to violate the first law of thermodynamics: Energy cannot be created or destroyed; it can only move into different forms. Because the product created through electron bifurcation has more energy than the material is starts with, it appears that the organism is creating energy out of thin air. However, this is not the case.
When enzymes catalyze reactions in a cell, they are fundamentally transforming energy. To engage in electron bifurcation, an enzyme takes a pair of electrons, splits them apart, sends the electrons down two different pathways, and, as a result, produces two separate compounds. One compound is a high-energy product, and the other compound is a low-energy product.
If you add up all the energy in the high- and low-energy products, the enzyme is not creating energy out of thin air, Lubner said. It is redistributing energy in a unique way to create something useful to the cell.
Lubner’s group at NREL developed an analogy to explain the concept more simply. An enzyme that uses electron bifurcation is like a child at dinnertime who must eat vegetables before getting dessert.
The child really wants dessert, which is high in energy and rich in calories and sugar, but he or she cannot eat it until after scarfing down the vegetables first, which are lower in energy and calories. So, like the enzyme, to get the delicious dessert (the high-energy product), children must give in and gobble down those veggies (the low-energy product).
A Universal Mechanism for Controlling Energy
Bifurcating enzymes—the enzymes that engage in electron bifurcation—are found in many different types of organisms. These enzymes can be found in photosynthetic bacteria, some plants, anaerobic organisms, and archaea, to name a few.
“The science community has known about electron bifurcation for a long time, and we have been able to observe the products that are formed, but understanding what the enzyme is doing, how it’s controlling the energy in this type of way, or the mechanism that it’s using, has been hotly debated over the years,” Lubner said.
In a nutshell, electron bifurcation is challenging to study. To analyze these systems more closely, Lubner’s team paired up with a theoretical chemist, David Beratan at Duke University, and used models, informed by experiments, to derive mathematical calculations to understand how energy flows within the enzyme.
The team discovered that, although there are many types of bifurcating enzymes in many different organisms, there is a universal mechanism for how the enzymes organize the way they control energy. For electron bifurcation to be efficient, energy levels within the enzyme must be poised in a certain way. For every electron that goes down the pathway that generates an energy-poor product, another electron always goes down the pathway to create an energy-rich product.
Bifurcating enzymes also conduct this mechanism in reverse. The enzyme sends the products in the opposite direction down their initial pathways. The products then converge at the bifurcating site and merge to create one product.
Lubner said observing the forward and reverse mechanisms changes how researchers think about the ways complicated chemical reactions are performed.
Future Renewable Energy Applications
In the future, Lubner’s team hopes to collaborate with materials researchers at NREL to design an artificial system that engages in electron bifurcation.
“Right now, all of the enzymes that utilize this mechanism produce compounds that are useful for a cell, things that we, as a society, are not going to take out of a cell and use as a fuel or a chemical,” Lubner said.
By designing an artificial system, they could utilize the features of electron bifurcation, such as efficient catalysis and high-level energy control, to make a biofuel or use it to reduce carbon dioxide (CO2). There is currently a strong interest for research on capturing CO2, a greenhouse-gas byproduct of industrial processes, and transforming it into something that is more useful.
Lubner recognizes these applied research goals are lofty. But she sees the potential in utilizing this research to help meet future energy goals.
“These enzymes are really unique in that they can control energy so well,” Lubner said, “and it’s not matched with what we can do artificially yet.”
Learn more about NREL’s bioenergy research.