Study Examines Lithium-ion Battery Electrodes through Advanced Microstructure Simulation
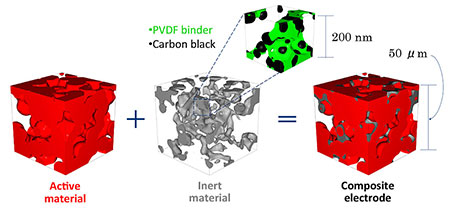
Increased driving range is one of the key challenges that must be overcome to boost market adoption of electric drive vehicles (EDVs). In addition to high energy density, batteries built for EDVs require high power density to perform effectively throughout the vehicle's lifetime.
Lithium-ion batteries, the EDV industry choice for a high capacity and lightweight package, must employ electrodes with a carefully balanced recipe to achieve both high energy and power density. Researchers at the National Renewable Energy Laboratory (NREL) and Texas A&M University have developed advanced computational models to enhance scientific understanding of a battery electrode's material recipe and how it controls battery performance.
In collaboration with Texas A&M, and with support from the U.S. Department of Energy's Vehicle Technologies Office and Computer-Aided Engineering of Batteries (CAEBAT) program, NREL Senior Energy Storage Engineer Kandler Smith contributed to the recently published study, Analysis of Long-Range Interaction in Lithium-ion Battery Electrodes.
The optimization of today's battery electrodes is entirely experimentally driven, making the design process both expensive and time-consuming. While computer models offer the potential to speed up the design process, most electrochemical models only capture the average behavior of a battery electrode. Under CAEBAT, Texas A&M, Argonne National Laboratory, and NREL have developed microstructure-scale electrochemical models that can more accurately predict the performance of battery electrodes based on their material recipe and manufacturing processes.
"To achieve high energy density, battery electrodes must pack in as much active material as possible, as the active material is what actually stores the energy. But for high power, electrodes must balance a mix of both active and inert materials," said Smith, stressing how the non-active materials can improve battery power but at the expense of energy density. For example, carbon black material dispersed in a polyvinylidene fluoride binder enhances electronic conductivity, while an open pore electrode structure allows for enhanced ionic conductivity by enabling the fast movement of ions when the electrode is filled with electrolyte. High power electrodes require both high electronic conductivity and high ionic conductivity.
As indicated in the paper, Smith and the Texas A&M team found that as additional non-active materials are added to an electrode design, the electronic conductivity increases. But with too much added, performance decreases due to the blockage of electrochemical active material surfaces and pores. The team explored a range of nickel-cobalt-manganese positive electrode designs, and the modeling results compared well to published experimental studies.
For this study and ongoing experimentation, Argonne has been conducting X-ray tomography with its Advanced Photon Source equipment to map the three-dimensional architecture of electrodes. Texas A&M has been extracting detailed geometry from the data and providing computational geometry to NREL. NREL is simulating the electrochemical response of electrodes, using the lab's high-performance computer. Moving forward, the team will continue to conduct modeling and experimental validation studies for a number of electrode designs, ultimately to improve the performance of Lithium-ion batteries and boost the market readiness of EDVs.
Learn more about NREL's battery modeling research for CAEBAT.
Last Updated May 28, 2025