To assess the value of wind energy to distribution, islanded, hybrid, and microgrid systems, the U.S. Department of Energy, its national laboratories, and industry collaborated on the Microgrids, Infrastructure Resilience, and Advanced Controls Launchpad (MIRACL) project. The 4-year MIRACL initiative evaluated how U.S. communities can safely, effectively, and efficiently integrate wind energy—as a distributed energy resource—into local grids.
The MIRACL project focuses on:
About
The U.S. Department of Energy Wind Energy Technologies Office launched the MIRACL project in 2018 in response to stakeholder feedback that information was needed to improve the operation, integration, and valuation of distributed wind energy in distribution, islanded, hybrid, and microgrid systems. Such information would build confidence that distributed wind energy could effectively complement—and, in some cases, compete with—other distributed energy resources.
MIRACL is part of a larger effort of the distributed wind energy research program to make wind energy an affordable, accessible, and compatible distributed energy resource. More individuals, businesses, and communities are turning to distributed energy resources for cost-effective, resilient energy, and the MIRACL project aimed to quantify wind energy's value to their grid systems. The initiative was led by the National Renewable Energy Laboratory (NREL) in collaboration with Idaho National Laboratory (INL), Pacific Northwest National Laboratory (PNNL), and Sandia National Laboratories (Sandia).
From 2018 to 2022, the MIRACL project supported the research and development of distributed wind energy technologies as well as the demonstration and validation of new tools, models, and potential capabilities for distributed wind energy systems. The project focused on developing innovative advanced turbine control capabilities, providing greater resilience, and improving valuation and power system modeling.
Research Areas
Valuation, Modeling, and Co-Simulation
Valuation, modeling, and co-simulation research and development accurately values grid system contributions from wind energy as a distributed energy resource.
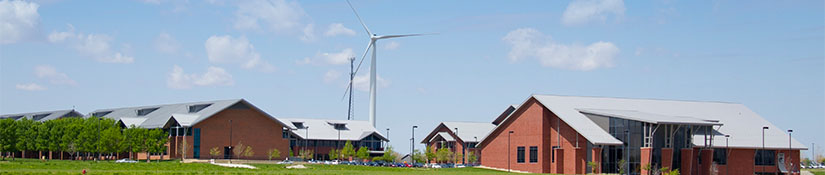
Heartland Community College in Normal, Illinois, uses power generated by a 1.65-MW Vestas wind turbine. Photo from Harvest the Wind Network
As communities deploy more distributed energy resources on their grid systems, a lack of accurate representation and valuation of wind energy in models and tools means the diversity benefits of wind energy, when combined with other distributed generation resources and storage, are not being realized.
Distributed wind energy generation can provide a variety of value streams, ranging from bulk energy services to societal impacts. The products developed as part of the MIRACL project can help stakeholders evaluate the benefits and cost of distributed wind energy and more accurately quantify its overall potential value for their unique energy systems.
To provide users, including community leaders and utilities, with more accurate information about distributed wind energy's value for microgrids, isolated grids, distribution networks, and hybrid power plants and systems, the team developed a valuation framework. This valuation framework is a modeling tool that quantifies benefits, such as avoided outage costs to customers.
The MIRACL team also improved power system modeling techniques within GridLAB-D and created a co-simulation platform to demonstrate how control and operation of distributed wind energy in microgrid applications could improve a system's resilience.
Distributed wind energy generation can provide a variety of value streams, ranging from bulk energy services to societal impacts such as energy resilience and more cost-effective energy systems. These values depend on ownership (e.g., utility- or community-owned facilities) and system configuration (e.g., whether the energy is distributed to individual homes or larger grid systems), among other factors. The tools developed through the MIRACL project will help stakeholders evaluate the benefits and costs of distributed wind energy and more accurately quantify its potential value for their unique energy system.
Output
The MIRACL team developed valuation, modeling, and co-simulation capabilities and tools, including the Value of Distributed Wind: A Valuation Framework, wind modeling improvements in GridLAB-D, the MIRACL Co-Simulation Platform for Control and Operation of Distributed Wind in a Microgrid, and the MIRACL Data Hub.
Value of Distributed Wind: A Valuation Framework provides a consistent, comprehensive approach to finding the net benefits of distributed wind energy. The framework considers typical use cases for distributed wind energy and accounts for a variety of stakeholders.
To improve the usability of the framework, the MIRACL team developed a Python-based service, available through GitHub, that allows users to better understand and optimize the value of a distributed wind energy project and identify to which stakeholders these values accrue. The service can calculate energy generation, avoided carbon dioxide emissions, demand charges, and reduction in on-peak load charges from generation and transmission utilities. With this framework, users can calculate values for grid-connected and local power systems and recognize hourly variations in prices. The service also has a unique co-optimization function; for mutually exclusive value streams, the service can weigh the relative values of each and display values for the optimal operational strategy. This analysis can inform power plant operators' operations strategies in addition to valuation exercises.
For example, an industrial facility manager could use the valuation framework to understand how added wind energy and storage could expand the value of an existing rooftop solar photovoltaic system. Wind energy could add value by addressing the facility's reactive power needs, expanding system resilience at a time of grid instability, and more.
The MIRACL team updated GridLAB-D to improve the flexibility and representation of distributed wind energy technologies in the tool's models. These updates can be accessed in GitHub on the develop branch of GridLAB-D.
GridLAB-D is an open-source tool that can simulate how each part of a distributed energy system might interact with every other part. The tool's algorithm can simultaneously simulate millions of independent devices—such as distributed wind turbines or energy storage systems—to understand how they could work together as a whole. A facility manager could use GridLAB-D to simulate how adding distributed wind energy to a system that includes photovoltaic panels and energy storage might affect the energy distribution. GridLAB-D can offer insights on energy distribution impacts over seconds or even years and during simulated disruptions caused by weather or other more unusual circumstances.
The MIRACL team built a co-simulation platform for researchers to test and validate capabilities of wind energy technology and facilitate the integration of their generated power in a seamless, plug-and-play manner into microgrids and distribution systems. It can also inform industry stakeholders looking to invest in resilience and efficiency improvements. The MIRACL co-simulation platform was developed as a basic platform that is easily customizable to accommodate stakeholders' particular software and hardware resources.
Microgrid operators can use this platform to evaluate distributed wind energy's value to a realistic microgrid. This platform is the only plug-and-play tool available for users to analyze the impact of distributed wind energy on their microgrid operating environment.
The MIRACL Data Hub is a centralized, accessible, and secure repository of information for the MIRACL project. The data hub, which houses wind turbine and microgrid data from multiple sources and locations, also supports other MIRACL research areas and is a critical tool for facilitating collaboration between the national labs and stakeholders.
The data hub collects three streams of wind energy data—two from the INL campus and one from NREL's. The hub's success demonstrates how live data streams from many devices and multiple locations can be aggregated in real time for research purposes. PNNL and INL are also collaborating to use data from the data hub for GridLAB-D simulations, which benefit from data collected from real wind turbines and microgrid systems.
INL hosts the data in a highly secure hub, which can be used for data analysis, distribution,
and storage. The data collection system is an extendable, straightforward relational
database server located in the INL High-Performance Computing infrastructure. To access
the data hub, users can request a high-performance computing account or contact Megan Culler.
Additional Resources
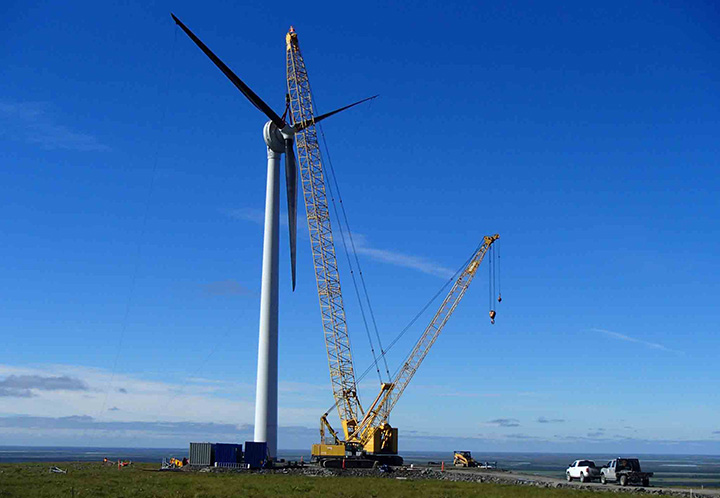
Valuation of Distributed Wind in an Isolated System, Energies (2021)
Valuing Wind as a Distributed Energy Resource: A Literature Review, Renewable and Sustainable Energy Reviews (2021)
MIRACL Co-Simulation Platform Lab Assets and Tools Integration (2022)
Distributed Wind Hybrid Microgrids With Autonomous Controls and Forecasting, Applied Energy (2023)
Advanced Controls
Advanced controls research and development demonstrates and documents the ability of advanced distributed wind turbine controls to support electric grid stability and resilience.
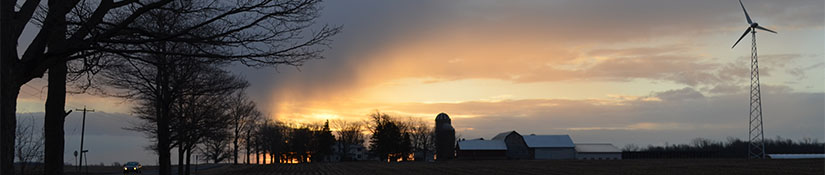
Advanced controls operate a wind turbine's electrical and mechanical systems using a network of sensors and external control commands. These controls can enable a wind turbine to support grid stability and maximize power production during disturbances, whether they be internal to the wind turbine, such as a fault, or external, such as a grid outage. This ability to react to events can reduce the chance of loads and generators tripping, lower the cost of wind energy, and facilitate more distributed wind energy integration with the grid.
The MIRACL project's advanced controls research explores how systems with high wind energy contributions can provide grid services to improve the resilience of the local power grid and generate revenue. Using high-fidelity simulations, the MIRACL team explored distributed wind energy integration in different use cases, including an isolated system, a grid-connected system, and a microgrid. The team also simulated ancillary grid services, such as inertia, frequency, and voltage support as well as active and reactive power control.
The team developed controls to mitigate internal wind turbine faults and examined how integrating energy storage into a wind turbine's electrical system could increase dispatchability (its ability to provide energy on demand). It examined how load control could support high contributions of distributed wind energy systems. And finally, the team demonstrated how distributed wind energy can work with a battery and photovoltaic array in a hybrid power system with integrated forecasting to maximize local resilience and participate in grid service markets.
The MIRACL project's models, publications, and use cases can help utilities, developers, and researchers understand various distributed wind energy capabilities. These references can help stakeholders understand which systems, including which advanced controls, might best serve their needs and their communities.
Output
For the MIRACL project, the team categorized projects into four use cases with similar technical, financial, market, and resilience requirements, challenges, and benefits. These include, for example, certification, valuation, cybersecurity, and fuel diversity.
The MIRACL high-level use cases for distributed wind encompassed wind turbines in:
- Isolated grids
- Grid-connected microgrids
- Behind-the-meter deployments (energy dispersed to individual homes or businesses)
- Front-of-the-meter deployments (energy dispersed to larger grid systems serving bigger communities).
For each use case, the team demonstrated, with high-fidelity simulation, how distributed wind energy can provide specific benefits in a variety of scenarios. The machine modeled was NREL's 600-kW controls advanced research turbine, and the microgrid models used are publicly available on GitHub. The team also developed models of small, distributed wind turbines with advanced controls (also available on GitHub), which will be installed at NREL's Flatirons Campus.
The team focused on the grid-connected microgrid use case. Using it demonstrated that microgrids could maintain local stability and maximize system economics—with all renewable power—by hybridizing distributed wind turbines and solar photovoltaics, autonomous device-level and system-level controls, battery energy storage systems with smart inverters, and forecasting. This includes riding through faults and grid transitions, handling changes in resource supplies, and participating in grid service markets. This demonstration can help microgrid operators understand how to integrate distributed wind energy into their all-renewable hybrid systems to increase system stability and value.
Controlling the load on the grid can support a stable grid frequency, which is particularly important for systems with high concentrations of renewable energy—especially those with more inverter-based resources and fewer traditional synchronous generators. In this literature review, the MIRACL team described various types of load-control systems and provided recommendations for future load control research and deployment. This review can help grid operators make informed decisions about how they control the load on grids with high concentrations of renewable energy.
Advanced Distributed Wind Turbine Controls Series: Part 1–Flatirons Campus Model Overview; Microgrids, Infrastructure Resilience, and Advanced Controls Launchpad (MIRACL) , NREL Technical Report (2022)
Advanced Distributed Wind Turbine Controls Series: Part 4–Wind Energy in Microgrids; Microgrids, Infrastructure Resilience, and Advanced Controls Launchpad (MIRACL) , NREL Technical Report (2022)
Demonstration of a Fault Impact Reduction Control Module for Wind Turbines, Wind Energy Science (2022)
Development and Validation of a Wind Turbine Generator Simulation Model, 54th IEEE North American Power Symposium (2022)
Integration of Storage in the DC Link of a Full Converter-Based Distributed Wind Turbine, NREL Technical Report (2022)
Microgrids, Infrastructure Resilience, and Advanced Controls Launchpad (MIRACL) Research: Controls , Department of Energy Fact Sheet (2021)
In 2021, a wind turbine emulator was permanently installed at Sandia's Distributed Energy Technologies Laboratory. The emulator—which mimics actual wind turbines at Sandia's Scaled Wind Farm Technology Site near Lubbock, Texas—offers an opportunity to study turbine plant behavior under multiple weather conditions and load demands. The wind turbine emulator consists of a scaled-down wind turbine motor and uses much of the same hardware and software that control actual turbines. The motor is connected to the lab's emulator system, allowing researchers to operate the virtual turbine under different conditions.
The team's wind turbine emulator will provide valuable data on how various wind power plant designs behave in different scenarios. Wind power plant owners and operators can then use this data to inform how they design their wind plants to maximize energy production and value.
Additional Resources
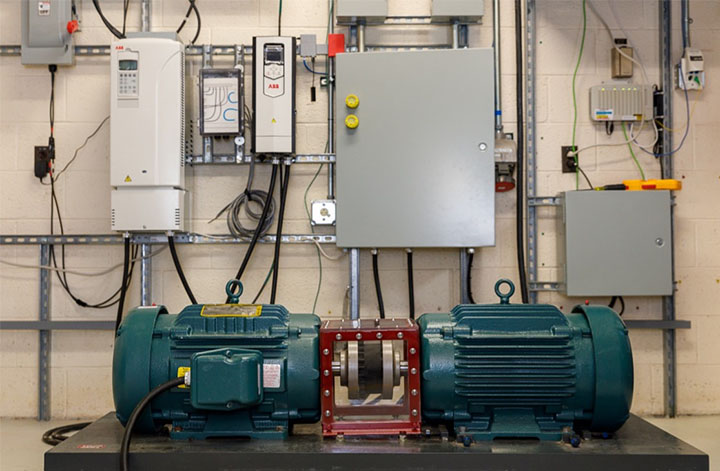
Advanced Distributed Wind Turbine Controls Series: Part 2-Wind Energy in Isolated Grids; Microgrids, Infrastructure Resilience, and Advanced Controls Launchpad (MIRACL) , NREL Technical Report (2022)
Advanced Distributed Wind Turbine Controls Series: Part 3-Wind Energy in Grid-Connected Deployments; Microgrids, Infrastructure Resilience, and Advanced Controls Launchpad (MIRACL) , NREL Technical Report (2022)
Development and Validation of a Wind Turbine Generator Simulation Model, 54th IEEE North American Power Symposium (2022)
Development of a Wind Turbine Generator Volt-Var Curve Control for Voltage Regulation in Grid Connected Systems , 54th IEEE North American Power Symposium (2022)
Distributed Wind Considerations, IEEE 1547-2018 Revision
Distributed Wind Hybrid Microgrids With Autonomous Controls and Forecasting (2022)
Load Control for Frequency Response—A Literature Review, NREL Technical Report (2021)
Model Characterization and Frequency Regulation in Wind-Diesel Hybrid Microgrids, 54th IEEE North American Power Symposium (2022)
Open Source Simulink Models for Eocycle, Bergey, and QED Distributed Turbines
Enhanced Resilience and Cybersecurity
Enhanced resilience and cybersecurity research and development achieves greater, measurable resilience in microgrids and distribution systems through distributed wind energy integration and cybersecurity.
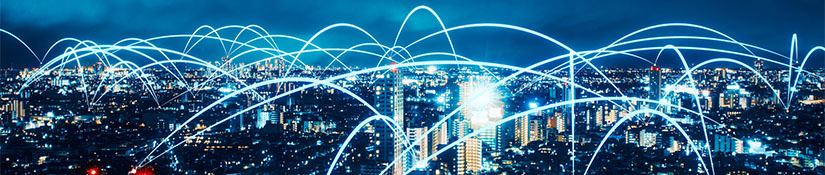
Variable energy resources, such as distributed wind energy, are often seen as detriments to power system resilience rather than benefits because they may not be available with the capacities or services required during a high-impact, low-frequency event—whether that is a physical threat, natural disaster, or cyberattack. However, distributed wind can be well suited to combat many resilience hazards if appropriate resilience goals and metrics are used. As climate change exacerbates extreme weather events and cyberattacks increasingly affect renewable resources, it is important to understand how distributed wind can be best used to enhance power system resilience, both on its own and as a component in hybrid energy systems.
As distributed energy resources are increasingly adopted, they pose an opportunity to provide renewable electricity generation to and improve the resilience of distributed grids, especially when included in hybrid power systems. Although distributed wind energy has the potential to improve grid resilience through participation in hybrid systems, distributed wind energy adoption is slower than that of other distributed energy resources.
The MIRACL team adopted a ground-up approach to explore how distributed wind energy could contribute to resilience on its own and in hybrid systems in various use cases, including behind-the-meter, front-of-the-meter, and off-grid applications.
First, the appropriate definition and metrics for resilience were considered. Next, a framework for planning and operating a resilient system with distributed wind energy was developed and tested. This framework was further developed into a user-friendly application. Finally, different approaches to enhance the resilience of distributed wind energy systems—including adding other resources to create hybrid systems, evaluating cybersecurity needs and challenges, and considering changes to control capabilities and methodologies—were explored.
The resources developed can help utilities, asset owners, manufacturers, and other stakeholders design and deploy secure distributed wind and hybrid energy systems that can enhance power system resilience in measurable ways beyond energy capacity and economic performance. Using the right metrics to evaluate resilience can help justify the cost of upgrading or installing a system by showing how the risks of natural, man-made, and cyber disasters can be mitigated.
Output
The MIRACL team demonstrated that distributed wind adds to overall system resilience when it is appropriately designed and deployed. The research showed not only how distributed wind can be used as a resilience asset but also how the use of distributed wind in hybrid systems and with advanced controls further enhances resilience beyond what any of the individual components could do alone.
The first challenge to evaluating how distributed wind could contribute to the resilience of power systems was a lack of standardized definition or metrics for resilience. Although it is a term increasingly used in the sector, it is often confused or conflated with reliability, which is a more standardized quality typically related to low-impact, high-frequency events.
After extensive literature review, the MIRACL team defined resilience for electric power systems as:
"a characteristic of the people, assets, and processes that make up the EEDS [electric energy delivery system] and their ability to identify, prepare for, and adapt to disruptive events (in the form of changing conditions) and recover rapidly from any disturbance to an acceptable state of operation."
Metrics to evaluate resilience using this definition were explored, specifically targeting those that are most applicable to systems with distributed wind. This new, standardized definition will make it easier for facility owners and operators to understand why resilience is valuable and identify strategies to enhance their systems' resilience.
Distributed Wind Resilience Metrics for Electric Energy Delivery Systems, INL Technical Report (2021)
To help system planners and operators design, operate, and improve the resilience of systems with distributed wind energy, the MIRACL team developed a resilience framework. The framework—which considers the specific characteristics, resilience goals, and hazards for a grid system—has been used to demonstrate ways distributed wind energy can add to the resilience of the system. The team's findings show that use of local, available generation resources and grid services, such as distributed wind energy, enhances system resilience.
Resilience Framework for Electric Energy Delivery Systems, INL Technical Report (2021)
After developing the process for resilience planning with distributed wind energy and documenting the use of the framework though individual case studies, the MIRACL team realized the value of automating and streamlining the planning stage of the resilience framework to make it more accessible.
The team developed a web application to do this, leveraging the Spine Toolbox platform and modeling capabilities, which allows users to quickly upload information about their power system, apply hazard simulations to the system, and analyze resilience metrics. This web application will make it easier for facility managers to quickly access the information they need to plan how to enhance their system's resilience.
Resilience Development for Electric Energy Delivery Systems (ResDEEDS): Resilience Analysis Application, INL Technical Report (2022)
As distributed wind energy grows, the attack surface and interest from adversaries also grow. It is important to ensure that cybersecurity is considered early in the design stage, with stakeholder interests considered, to avoid the burden from becoming untenable. The distributed wind energy industry is not currently motivated to prioritize cybersecurity. However, best practices, which could effectively address some of the biggest risks, are not expensive to implement, and disseminating cost-effective practices to relevant stakeholders can help communities advance their energy portfolios and secure their path to net-zero carbon emissions.
The MIRACL team developed guidance to explain the cyber architecture of distributed wind energy and the role of various stakeholders. And, to incentivize investment in cybersecurity, the team created a cyber risk management architecture that explains the risks cyberattacks pose to a business. These resources can help the distributed wind energy industry understand the long-term value of incorporating cybersecurity measures into their planning at an early stage of development.
Cybersecurity Guide for Distributed Wind, INL Technical Report (2021)
A Cyber-Resilience Risk Management Architecture for Distributed Wind, Resilience Week (2021)
The MIRACL team developed ways to quantify and demonstrate the incremental value distributed wind energy can provide in hybrid power systems through a series of analyses and case studies. In one report, Stronger Together: The Value Impacts of Hybridizing Distributed Wind, it identified value streams enhanced by hybridization of distributed wind energy, and in another publication, Quantifying Technical Diversity Benefits of Wind as a Distributed Energy Resource, it developed metrics that quantified the resilience value of hybridization of distributed wind energy. It also presented the areas in the United States that can best leverage these technical benefits through complementary resources, determined via national hybrid resource analysis, in the Wind and Solar Hybrid Power Plants for Energy Resilience technical report.
The team demonstrated the technical benefits of hybridization, particularly for resilience value, in case studies by integrating the project's frameworks—the resilience framework, the Hybrid Optimization and Performance Platform, and the valuation tool. In the Isolated Grids and Grid Connected Turbine Reference Systems Report, the report's authors demonstrated these frameworks on distributed grids in the village of St. Mary's in Alaska and the Iowa Lakes Electric Cooperative in Iowa. The authors then leveraged these integrated frameworks to develop a new method for designing hybrid power systems to maximize resilience. It demonstrated this capability on a distributed grid in Algona, Iowa, in Hybrids for Resilience: A Case Study in Algona, Iowa.
All this work will help facility managers better understand the value that distributed wind energy can provide to hybrid power systems and make informed decisions about how and where to integrate wind energy into theirs.
Fiscal Year 2021 Isolated Grids and Grid-Connected Turbine Reference Systems, NREL Technical Report (2022)
Hybrid Distributed Wind and Battery Energy Storage Systems, NREL Technical Report (2022)
Hybrids for Resilience: A Cast Study in Algona, Iowa (2022)
Quantifying Technical Diversity Benefits of Wind as a Distributed Energy Resource, IEEE Power & Energy Society Innovative Smart Grid Technologies Conference (2020)
Stronger Together: The Value Impacts of Hybridizing Distributed Wind, The Electricity Journal (2022)
Wind and Solar Hybrid Power Plants for Energy Resilience, NREL Technical Report (2022)
Additional Resources
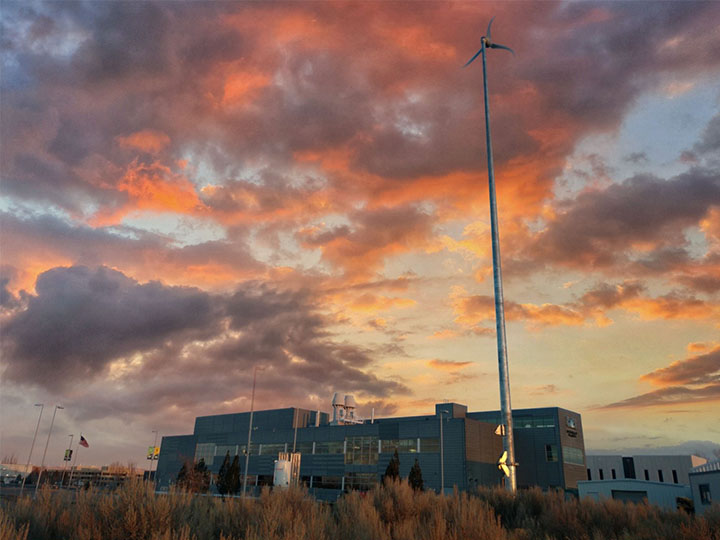
Case Study: Applying the INL Resilience Framework to Iowa Lakes Electric Cooperative Distributed Wind Systems , INL Technical Report (2022)
Case Study: Applying the INL Resilience Framework to St. Mary's, Alaska, INL Technical Report (2022)
Case Study: Resilience Benefits of Distributed Wind Against Fuel and Weather Hazards in Alaska , IEEE Power Engineering Society General Meeting (2022)
Cybersecurity for Distributed Wind: What Manufacturers Need To Know, INL Technical Report (2021)
Cybersecurity for Distributed Wind: What Integrators Need To Know, INL Technical Report (2021)
Cybersecurity for Distributed Wind: What Operators Need To Know, INL Technical Report (2021)
Electrical Grid Resilience Framework With Uncertainty, Electric Power Systems Research (2020)
Resilience in an Evolving Electrical Grid, Energies (2021)
The Virtualized Cyber-Physical Testbed for Machine Learning Anomaly Detection: A Wind Powered Grid Case Study , IEEE Access (2021)
Future Work
Although the MIRACL project has concluded, participating labs continue to use the initiative's capabilities and collaborations in other research projects.
The 2022–2023 On-Site Wind for Rural Load Centers project will be led by INL, with NREL and PNNL collaborating. This multilab research team will study the energy and resilience needs of rural load centers, which move electricity from a source, such as a wind turbine, to a destination. Such load centers have strong distributed wind energy potential.
The project team plans to develop a toolkit, including design templates, valuation strategies, energy equity analysis, and resilience boosters for hybrid power systems as a way to streamline and encourage adoption of distributed wind energy.
Project contact: Megan Culler, INL
The Wind Hybrid Integration Platform (WHIP) project is a 3-year, Sandia-led project intended to develop a novel distributed energy resource management system that enables the integration of high penetrations of wind turbine generators with other distributed energy resources.
The proposed control system, WHIP, will measure power system parameters to estimate the system's status, forecast its state over a short-term horizon, and issue optimal set-point commands to distributed energy resources to regulate voltage and improve the system. The WHIP will also allow plug-and-play functionality for different distributed energy technology types, such as wind turbines, photovoltaics, and energy storage systems. The team also plans to account for mechanical issues related to power curtailment through a one-of-a-kind solution that integrates an advanced wind turbine generator into wind and hybrid power system distributed energy resource management system controls.
Project contact: Rachid Darbali-Zamora, Sandia
For updates on distributed wind energy research and activities across the labs, please visit the U.S. Department of Energy Wind Energy Technologies Office.
Partners and Collaboration
The MIRACL project benefited from many partnerships and collaboration with:
- Algona Municipal Utilities
- Alaska Village Electric Cooperative
- Iowa Lakes Electric Cooperative
- Iowa State University
- General Electric
- Midcontinent Independent System Operator
- QED Wind Power
- Eocycle Technologies Inc.
- Bergey Windpower Co.
- Natural Resources Canada
- National Rural Electric Cooperative Association
- Renewable Energy Alaska Project
- Energy Reform
- SWG Energy Inc.
- Alaska Center for Energy and Power
- Tom Wind
- Enernex.
Share
Last Updated Feb. 19, 2025