New Insights Revealed Through Century-Old Photochemistry Technique
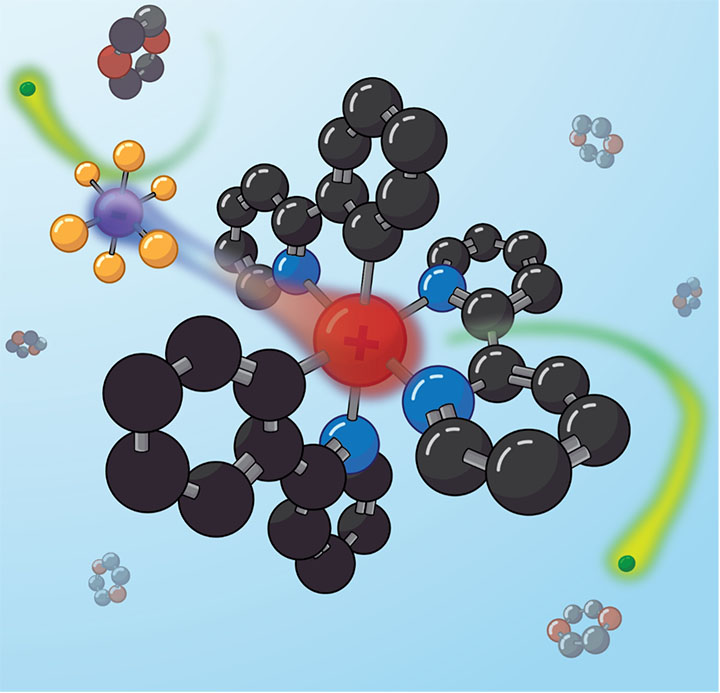
As the poet Dylan Thomas once explained, it is "the force that through the green fuse drives the flower."
Organic photochemistry brings life to Earth, allowing plants to "eat" sunlight. Using this power of light to make new molecules in the lab instead of the leaf, from fuel to pharmaceuticals, is one of the grand challenges of photochemical research.
What is old is new again. Sometimes gaining new insight requires a return to old tools, with a modern twist. Now, a collaborative team from the National Renewable Energy Laboratory (NREL) and Princeton University has resurrected a century-old microwave technique to reveal a surprising feature of well-established light-driven chemistry.
Published in Nature Chemistry, "Ion-pair reorganization regulates reactivity in photoredox catalysts" documents the work led by NREL Chemistry and Nanoscience Fellow Garry Rumbles, Research Professor Obadiah Reid, and University of Colorado Boulder graduate student Justin Earley. The work was carried out as part of the Renewable and Sustainable Energy Institute (RASEI), a joint institute between NREL and the University of Colorado Boulder.
This work, which is part of a U.S. Department of Energy (DOE) Energy Frontier Research Center titled Bioinspired Light-Escalated Chemistry (BioLEC) centered at Princeton University, reveals how an important class of "photoredox catalysts" operates, leading to unforeseen ways to manipulate their efficiency and selectivity.
Photoredox catalysis is a branch of photochemistry that has made a comeback in recent years, using light rather than heat or highly reactive chemicals to drive chemical reactions. This makes it possible to drive reactions with high kinetic or thermodynamic barriers with better control over the final product and potentially less harmful ingredients.
However, to use these new reactions at the manufacturing scale, a detailed understanding of how the reaction works is required.
"We started this work in order to learn how charges move during light-driven reactions," Reid said. "But we never expected the first catalyst we studied would surprise us like this."
The catalyst studied by the NREL team was an ionic compound composed of two halves. One half was positively charged, and the other was negative, so that the overall charge added to zero. In this case, the positive side was thought to do all the work in light-driven chemical reactions, while the negative side was a supposedly inert "counterion."
"We found that the counterion actually moves when the catalyst is excited by light, and that it can block certain kinds of reactions," Reid said. "Like a linebacker, sometimes your job is to just get in the way. That's just what the counterion turns out to do."
By measuring exactly how their microwave signal changed as it passed through the solution—with and without light—the team showed that the negatively charged counterion moved after the molecule was excited with light. "This matters," Earley said, "because molecules need a path for electrons to move through in order to initiate a chemical reaction, and the counterion can block it." The team discovered that the blocking action of the counterion led to a factor of four change between two different kinds of reactions.
Rumbles said that although the measurement tool used by the NREL team has been around for over 100 years, scientists historically had to conduct time-consuming control experiments to interpret their results. Computers changed that. Instead, the NREL team used quantitative simulations of how the catalyst molecules rotate in solution to help interpret their results.
Research funding was made possible by the DOE Office of Science as part of a BioLEC Energy Frontier Research Center grant awarded to Princeton University under grant number DE-SC0019370.
Last Updated May 28, 2025