A Window Into the Future of Wave Energy
Award Helps Move Cost-Effective, Productive, Robust Wave Energy Design a Step Closer to Commercialization and Widespread Use
In 1974, Stephen Salter, a professor at the University of Edinburgh, sent his “ducks” into the Scottish seas, launching the world’s first major wave energy project. But the ocean’s rough heaves and surges proved too much for his house-sized, floating generators. Like the more recent Pelamis’ P-750 model and Aquamarine’s Oysters, they succumbed to the power they were meant to harness.
“We have to ask ourselves,” said Krish Thiagarajan Sharman, the endowed chair in renewable energy at the University of Massachusetts Amherst, “why have we been working on this for so long? Why don’t we have grid-ready, commercial-scale wave energy systems out in the world?”
The answer: Wave energy technology needs to be cheaper, produce more energy, and brave the ocean’s brawn better and for longer.
Now, the marine energy team at the National Renewable Energy Laboratory (NREL), has designed a system that could achieve all three needs. The variable-geometry, oscillating, surge wave energy converter creates windows for waves to pass through so wave energy devices don’t bear the full force of their power. The design could also be more cost-effective, productive, and resilient.
Two years ago, that concept earned a competitive award from the U.S. Department of Energy’s Technology Commercialization Fund (TCF), a nearly $30-million funding opportunity designed to help promising, high-impact energy technologies move toward commercialization. With the TCF award’s critical support, Nathan Tom, a mechanical engineer at NREL, partnered with Sharman. Together, they moved the wave energy converter system from theory to practice, nudging the potential solution closer to commercialization and wave energy closer to widespread deployment.
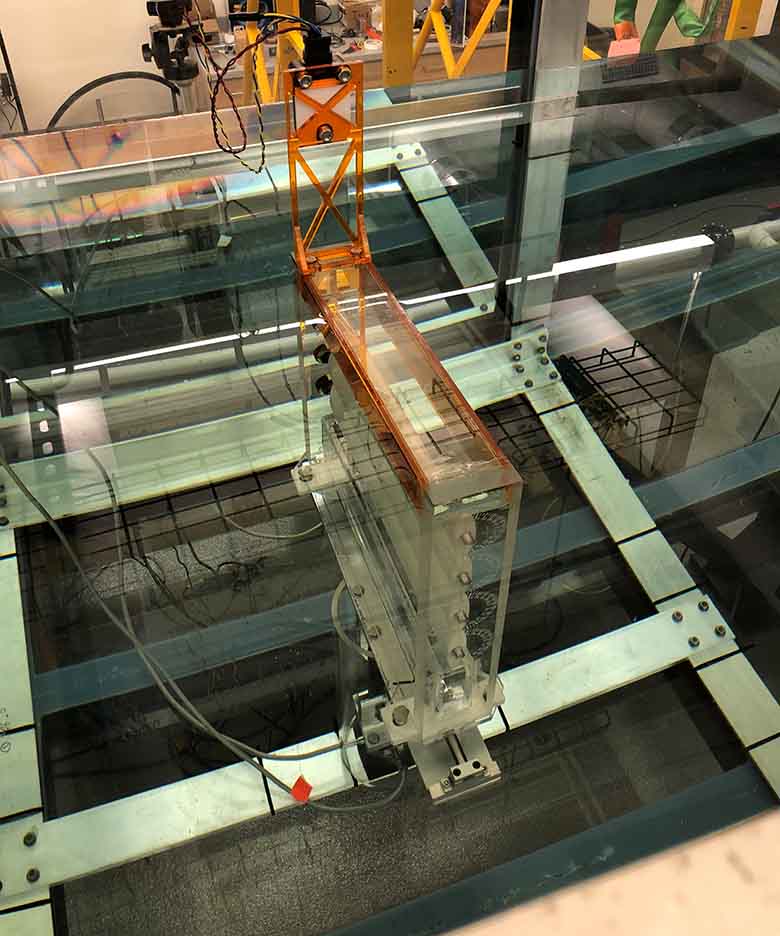
“All wave energy devices need a way of surviving for several years in the ocean,” said Sharman. “This is one way.”
Wave energy might not match the global power production of wind and solar energy anytime soon, but it’s still a critical source of clean, renewable energy. Waves are more predictable and reliable than solar or wind energy, and they could power hard-to-reach locations, like coastal communities and remote islands, which currently depend on expensive, carbon-intensive diesel imports. Wave energy devices could also power offshore fishing, marine research, or military operations that need to reach deeper waters. In the United States, waves carry the equivalent of about 80% of the country’s energy needs. Not all that energy can be practically harnessed, but the industry could access enough to ease the country’s transition to 100% clean energy.
Today, wave energy isn’t powering much—yet. Today’s designs falter for the same reason Salter’s ducks struggled; the ocean packs power stronger than any winds, and while some recent wave energy inventions are outfitted with steel hulls, this armor is too heavy and expensive to make the technology viable. Today, about 35%–50% of wave energy costs are spent on structural enhancements.
“Our systems are just too heavy,” Tom said. “To withstand really extreme loads, orders of magnitude higher than when the device is operating and producing power, they’re built with too much steel. We need to start thinking outside the box.”
NREL’s design is certainly outside the steel box. To source electricity from waves, the more-cost-effective, lighter, more-robust wave energy converter uses a rectangular paddle that sways back and forth on a bottom hinge, like a cattail in the wind. The swaying paddle is a common design feature for wave energy devices. But this one is far more adaptable. As waves go from productive to destructive, the device’s operator can open one or more horizontal flaps, creating gaps for that violent energy to escape.
This extra level of control not only protects the device, it could also help the converter produce more energy. When seas change, remote operators can toggle each flap to extract as much energy as possible.
Using the funding from the TCF award, Tom and Sharman built their first small-scale prototype to test in the university’s wave tank. The project team included University of Massachusetts Amherst graduate student Jacob Davis and postdoctoral scholar Jessica Nguyen (Nguyen started out as a graduate student). Cole Burge and Salman Husain, National Renewable Energy Laboratory undergraduate and graduate research interns, also contributed.
"The variable geometry project has had a tremendous influence on my career trajectory,” said Davis. “In fact, this experience spurred my passion so much, that the focus of my present Ph.D. work is entirely on the measurement and understanding of ocean surface waves. I look forward to a career spent applying this knowledge to a broad range of ocean-related challenges, including the role of waves in marine energy conversion, weather, and climate."
Together, the team checked their theoretical models against experimental data and found, to their relief, that the models accurately predicted the device’s performance. That was a promising start. But the design needs to go through far more iterative steps before it can reach the ocean.

“I could quickly design something to go in the water,” said Tom, “but what if it only produces 1 watt? It’s going to cost me a lot of money—boats, divers, pulling a cable from sea to land—which is not cheap.”
That “big move,” said Tom, which comes with large expenses and high risk, makes potential wave energy industry partners wary of investing in even highly promising designs, like their variable-geometry prototype. Many tend to wait for concepts to prove their success in low-risk environments, like numerical models and wave tanks.
But that progressive work requires funding, too, which is why TCF awards are a critical bridge from theory to market. “We want to develop a patented technology far enough where industry thinks, ‘OK, this is a good idea. That’s something I want to try to incorporate into my design,’” said Tom. “That’s when we’ll transfer it over to industry. And for that, the TCF award is super helpful.”
The award also allowed the team to test another novel aspect of their design: a raised foundation. Often, oscillating surge wave energy conversion devices are fixed to the seabed where swirling debris and sand can interfere with operation. Lifted off the seafloor on a column, the device can avoid those obstacles and access a greater number of locations between sand and surface or in deeper waters. Because surface waters are often more energetic, devices that can reach those areas could produce more power.
Together, the raised foundation and variable-geometry could help wave energy devices adapt to their ocean environment better than previous designs, including Salter’s ducks (though these early forays were critical to moving the industry forward).
“We look at waves not as a force to reckon with,” said Sharman, “but to use to harness energy. It’s a very holistic, symbiotic approach to manage our interaction with the ocean.”
Learn more about NREL's variable-geometry marine energy project and water power research. Subscribe to the NREL Water Power newsletter for NREL's latest on marine energy.